Peak Phosphorus: the sequel to Peak Oil
Professor Stuart White
Director, Institute for Sustainable Futures, University of Technology Sydney (UTS) Australia
Dr Dana Cordell
Research Principal, Institute for Sustainable Futures, University of Technology Sydney (UTS) Australia
Note:
This information is based on a peak phosphorus analysis first undertaken by Professor White at the Institute for Sustainable Futures, University of Technology Sydney in 2006 (published in Cordell, Drangert & White, 2009). The peak phosphorus curve has been updated since IFDC released a revised estimate of phosphate reserves in 2010 (figure 1). Several other peak phosphorus studies by other authors have now been undertaken since global reserve data was updated in 2010, indicating a peak year between 2035 and 2075 (e.g. Mohr & Evans 2013 and Walan 2013).
1. Introduction
All modern agricultural systems are dependent on continual inputs of phosphate fertilizers derived from phosphate rock. Yet this is relying on a finite resource and demand for phosphorus could outstrip supply in the next 30-60 years in no changes are made to the current system.
As highlighted by Hubbert first in 1949 (Hubbert, 1949), production of oil resources will eventually reach a maximum rate or ‘peak’ based on the finite nature of non-renewable resources, after which production will decline. Hubbert and later others contest that the important period is not when 100% of the resource is depleted, but rather when it reaches a production maximum, which occurs when 50% of the resource is still in the ground. After this point, production decreases, placing upward pressure on prices and increasing international tensions (Campbell, 1997). While the exact timing may be disputed, it is clear that already the quality of remaining phosphate rock reserves is decreasing and cheap fertilizers will be a thing of the past. Like oil in the 1970’s, phosphate rock is experiencing it’s first significant price shock – a 700% increase from US$50/tonne to US$350/tonne in just 14 months (Lewis, 2008).
Yet there are no alternatives to phosphate rock currently on the market that could replace it at any significant scale. While various small-scale trials are being undertaken, commercialization and implementation on a global scale could take decades to develop.
2. Methodology and analysis
This analysis of peak phosphorus is based on estimated P in current world phosphate rock reserves (approximately 2358 MT P ) based on US Geological Survey data and cumulative production between 1900-2007 (totaling 854 MT P) based on US Geological Survey data (Buckingham and Jasinski, 2006; Jasinski, 2007, 2008) and European Fertilizer Manufacturers Association (2000). The area under the Hubbert curve must equal the depleted plus current reserves, totaling approximately 3,212 MT P. Units of phosphorus are presented as elemental P, rather than P2O5 (containing 44% P) or phosphate rock (containing 29-34% P2O5) as commonly used by industry.
The data for production is fitted using a Gaussian distribution (Laherrere, 2000), based on the depleted plus current reserves estimate of 3,212 MT P, and a least squares optimisation which results in a production at peak of 28 MT P/a and a peak year of 2034 (figure 1).
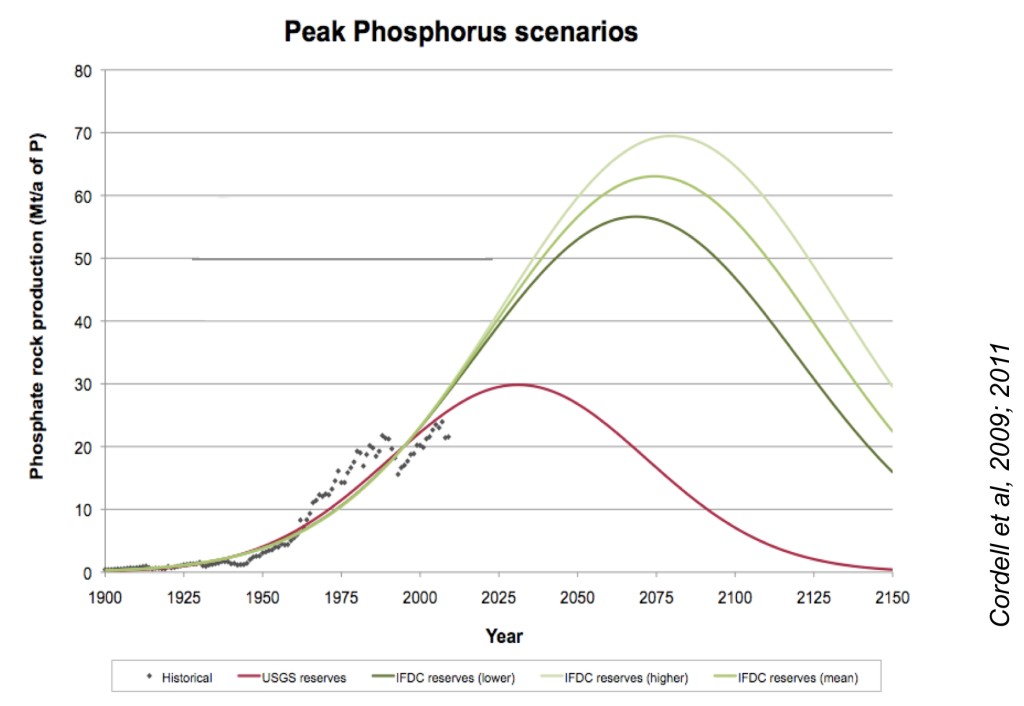
The actual timing may vary due to production costs (such as price of raw materials like oil), data reliability and changes in demand and supply.
In 2007 the Energy Bulletin posted a peak phosphorus article by geologist Patrick Déry and co-author Bart Anderson (Déry and Anderson, 2007). In this article, the authors estimate U.S phosphate rock reserves peaked almost 20 years ago, around 1989. This same article suggests global reserves peaked around the same year. Whilst there was indeed a production peak in this year, like oil peaks in the 70’s, this observed peak was not a true maximum production peak, and was instead a consequence of political factors such as the collapse of the Former Soviet Union and decreased fertilizer demand from Western Europe. According to USGS staff, Moroccan and Western Saharan reserves, which account for a significant proportion of today’s global production, are currently being mined at a relatively constant rate that is less than the maximum production (USGS, pers comm. 5/9/07).
3. Comparing Peak P and Peak Oil
While it is understood that phosphate rock, like oil and other key non-renewable resources will follow a Hubbert production curve, a key difference between peak oil and peak phosphorus, is that oil can be replaced with other forms of energy once it becomes too scarce. Where as there is no substitute for phosphorus in food production (Cordell, et al, 2009). P cannot be produced or synthesized in a laboratory. Quite simply, without phosphorus, we cannot produce food.
A second key difference is that oil is unavailable once it is used. While phosphorus is an element that can be captured after use and recirculated for use within economic and technical limits.
Peak phosphorus is also linked to peak oil. For example, the recent oil price shock and growing concern about climate change has stimulated a dramatic increase in biofuel crop production globally, which in turn increases the demand for phosphate fertilizers, and hence the proximity of the phosphorus peak.
A more detailed comparison of peak oil and peak phosphorus is provided in Cordell and Kerschner (2007).
4. Sceptics of the ‘Hubbert’ Curve
Peak Oil sceptics commonly argue that the market will take care of things: that resource scarcity is ‘relative’, and one scarce resource can simply be replaced by another indefinitely, because as price rises, investment in new technology will always improve efficiency of extraction and use (Steward et al, 2005). This is the basis of the market system – neoclassical economic theory – which does not acknowledge the finite nature of non-renewable resources like phosphate rock (or oil). This means that the concepts of Peak oil and Peak Phosphorus are not supported by the market system. (see Cordell and Kerschner for 9 Reasons why markets fail to manage global P resources for food production). Other sceptics don’t deny that peaks will one day occur, rather they dispute the timeline and insist a peak is more in the distant future (Caveny, 2006).
While the Hubbert peak has been hotly contested since it’s conception almost 60 years ago, it is increasingly gaining traction as the price of oil shoots well beyond US$100/barrel. In November 2007 the then International Energy Agency Chief Economist stated in a noteworthy interview: “if we don’t do anything very quickly, and in a bold manner, our energy system’s wheels may fall off – within the next seven years” (Financial Times, 2007).
5. Future management of P
While the recent price spike in phosphate rock is likely to trigger further innovations in and adoption of phosphorus recovery and efficiency measures, the market alone does not have enough adaptive capacity to manage phosphorus in a sustainable, equitable and timely manner in the long-term.
We are entering a new and unprecedented era of global environmental change this Century. As we are learning from climate change and global water scarcity, a long-term time frame is essential for understanding, managing and adapting our current system in a timely way. The same applies to global food security and phosphorus resources. The time frames of the market, and key actors in the market system (like the fertilizer industry) are typically short term, dealing with 5-10 year horizons at the most, rather than 50-100 year time frames that are required. Whilst there is not enough reliable data today to predict the exact year peak phosphorus will occur, what is clear is that discussion on alternative phosphorus sources and governance models is required now to ensure that the world’s farmers have sufficient access to phosphorus fertilizers in the long-term to feed humanity, without compromising the environment, livelihoods and economies.
A balanced diet results in depletion of around 22.5kg/yr of phosphate rock per person based on current practice. This is 50 times greater than the 1.2 g/person recommended daily intake of elemental P (Cordell, Drangert and White, 2009). The current system of mining and processing phosphate rock, international transport and storage, fertilizer application, harvest, food processing, retailing, storage and final consumption is inefficient and presents many opportunities for both increasing efficiency throughout the system, and for capturing used phosphorus in human and animal excreta and food and crop residues.
References
ASPO (webpage), The Association for the Study of Peak Oil and Gas, www.peakoil.net
Buckingham, D.A and Jasinski, S. M. (2006) Phosphate Rock Statistics, Historical Statistics for Mineral and Material Commodities in the United States, Data Series 140 US Geological Survey minerals.usgs.gov/ds/2005/140/
Caveny, R. (2006), Global Oil Production About To Peak? A Recurring Myth, World Watch Magazine, January/February 2006, Volume 19, No. 1 pp. 13-15. www.worldwatch.org/epublish/1/v19n1
Campbell, C.J. (1997), Better understanding urged for rapidly depleting reserves, Oil & Gas Journal; Apr 7, 95, 14.
Cordell, D. and Kerschner, C. (2007), Governing Global Resource Peaks: the case of peak oil and peak phosphorus, 1st version prepared for the Institutional Analysis of Sustainability Problems proceedings book, June 2007, Marie Curie Emerging Theories and Methods in Sustainability Research series, Bratislava.
Cordell, D. and Kerschner, C. (2008), 9 Reasons why markets fail to manage global P resources for food production, The Story of P Information Sheet 3, Institute for Sustainable Futures, University of Technology, Sydney (UTS) Australia and Department of Water and Environmental Studies, Linköping University (LiU) Sweden. [Download PDF]
Cordell, D., Drangert, J-O. and White, S, The Story of Phosphorus: Global food security and food for thought,Global Environmental Change Journal, (2009), doi:10.1016/j.gloenvcha.2008.10.009.
Déry, P. & Anderson, B. (2007) Peak phosphorus. Energy Bulletin. energybulletin.net/node/33164
EFMA (2000), Phosphorus: Essential Element for Food Production, European Fertilizer Manufacturers Association (EFMA) Brussels.
Financial Times (2007) Interview with Faith Birol, Chief Economist of the International Energy Agency (IEA), 7th November 2007, London.
Hubbert, M. K. (1949) Energy from fossil fuels. Science, 109, 103.
IFA (2006) Production and International Trade Statistics, International Fertilizer Industry Association, Paris www.fertilizer.org/ifa/statistics/pit_public/pit_public_statistics.asp
Jasinski, S. M. (2008), Phosphate Rock, Mineral Commodity Summaries, U.S. Geological Survey, January 2008 minerals.usgs.gov/minerals/pubs/commodity/phosphate_rock/
Jasinski, S. M., (2007), Phosphate Rock, Mineral Commodity Summaries, , U.S. Geological Survey minerals.usgs.gov/minerals/pubs/commodity/phosphate_rock/
Laherrere, J.H. (2000) Learn strengths, weaknesses to understand Hubbert curve, Oil & Gas Journal, 98, 16, p63.
Lewis, L. (2008) Scientists warn of lack of vital phosphorus as biofuels raise demand, The Times Online, 23rd June, 2008 www.timesonline.co.uk/tol/business/industry_sectors/natural_resources/article4193017.ece
Stewart, W., Hammond, L. & Kauwenbergh, S. J. V. (2005) Phosphorus as a Natural Resource. Phosphorus: Agriculture and the Environment, Agronomy Monograph No.46. Madison, American Society of Agronomy, Crop Science Society of America, Soil Science Society of America.